Your Black Hole Questions Answered
April 30, 2019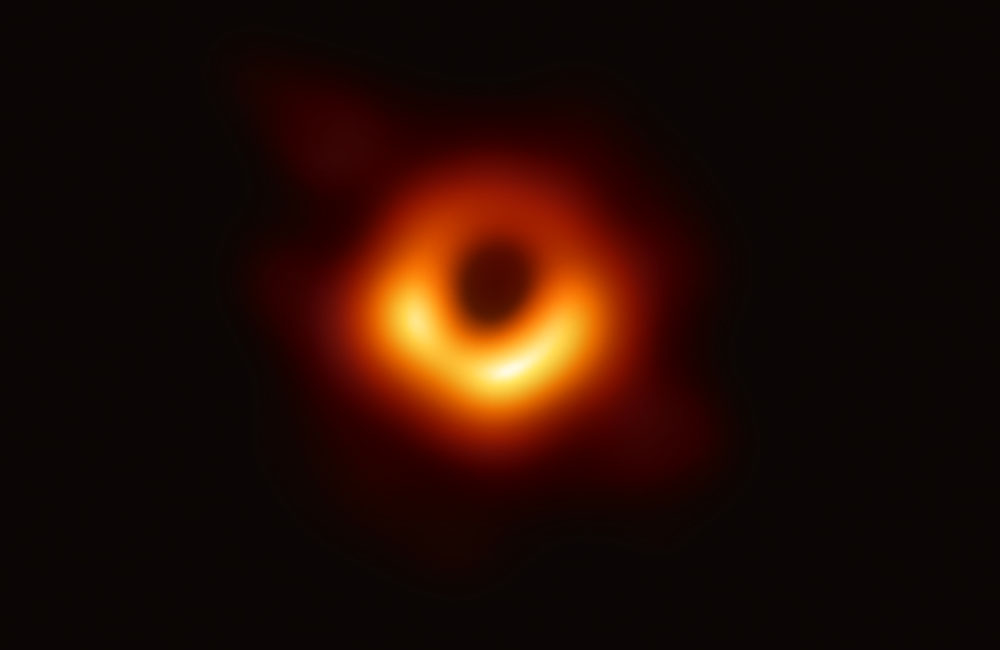
A couple of weeks ago, after the release of the first image of a black hole, we asked our social media followers to submit their questions about the phenomenon. Here are some answers, supplied by members of the Harvey Mudd College physics community.
@sauteractual asked via Instagram, how frequently do black holes occur and do they ever occur within our own galaxy or just neighboring galaxies?
Professor of physics and dean emeritus Tom Helliwell answers:
There is a supermassive black hole at the center of our galaxy as well, with a mass of about 4 million times that of our Sun. This is a large mass, but not nearly so large as that of the recently imaged black hole at the center of M87, which has a mass of 6.5 billion solar masses.
Our central black hole, which is located in the direction of the constellation Sagittarius, is also not nearly as active as the one in M87, in that ours has less hot gas and fewer stars swirling around it. We believe there are also a very large number of black holes scattered through our Milky Way galaxy that originated from the cores of large stars which collapsed when the stars underwent supernova explosions. The outer portion of such a star blows up, forming a supernova that can be as bright as the rest of the galaxy put together, at least for a few days, after which it quickly dims. In the meanwhile, the core of such a star collapses, to become a so-called “neutron star” if it is not too massive, or a black hole if it is sufficiently heavy.
We have not observed a supernova explosion in the Milky Way galaxy itself since the year 1604, but when our galaxy was younger, billions of years ago, there would have been more frequent supernova explosions, some of which undoubtedly led to black holes. These black holes would still be circulating around in our galaxy. There are likely thousands or even millions of such black holes in our galaxy, with masses of a few times that of our Sun; the number of them is unknown. In any case, it is extremely unlikely that our solar system will meet up with one in the foreseeable future, at least we can sincerely hope so.
Another Instagram follower, @Ptowncracker, asks these questions answered by physics professor Brian Shuve:
Is there a way around it to possibly look at the other side?
You can certainly see “around” the black hole—any light that passes directly through the black hole will get stuck in it and won’t come out, but light from sources behind the black hole might be bent around the black hole due to the curvature of spacetime: This is an effect called gravitational lensing. The actual “hole” on M87 is relatively small compared to the size of the galaxy, so we can see most objects behind the black hole.
What happens when you go into the black hole?
The point of no return is called the “event horizon”—any light, or other matter, that gets closer than the event horizon can’t come out again. However, according to general relativity, nothing special actually happens at the event horizon—if you were to pass through, you wouldn’t necessarily know you had gone through. This is because gravity is a manifestation of curved space, but if you zoom into a curved space, it appears to be flat. For example, if you look out your window, you might think the Earth is flat because you are so close (and small compared to the size of the planet) that it looks flat, even though if you zoom out enough you can see that it is curved. Your body is small compared to the typical curvature of space, and so if you are freely falling you don’t feel anything different.
As you get closer to the center of the black hole, however, the space becomes so curved that the space near your feet is curved differently from the space near your head. This leads to what are called “tidal forces” (because they are responsible for the ocean’s tides, among other things) that will essentially rip you apart. Your body would be elongated until your tissues are no longer able to keep your legs connected to your body. Gruesome perhaps, but this only happens once you get very close to the black hole center.
What is on the other side? Where does the hole go—to another dimension?
The answer is: We don’t quite know. According to the theory of general relativity, there is no “other” side—things just get crushed at the center of the black hole. However, the curvature of space time at the center of the black hole is technically infinite, and the underlying assumptions of our theory break down at this point. It means it’s possible that something very different happens near the very center than what I’ve just described. What we do know (and that this photo confirms) is that nothing comes out of the black hole, so whatever happens to objects once they cross the event horizon, there’s no way for us to know what happens for sure!
@preet_h.i asks via Instagram, can the Hawking radiation theory be proved by the data collected? Thus do black holes shrink over time?
We asked physics professor Vatche Sahakian to tackle this one.
Very unlikely. The temperature of a black hole is lower the larger the black hole, and it is extremely low for supermassive black holes. The rate of evaporation is correspondingly very slow. A black hole with the mass of our Sun would take many, many times the age of the universe to evaporate away (ten to the power 58 times the age of the universe!). A supermassive black hole with millions of solar masses is even slower to evaporate away. So, black hole evaporation is really unlikely to be directly detected. But then, by imaging this black hole, they were essentially able to resolve the lettering on a coin over the distance between LA and New York, so who knows what the future holds …
In answer to @rickeleine’s questions: Which is more tangible: black holes or love?, Sahakian gives a very diplomatic physicist’s answer:
Love of black holes is the most tangible of all. Argument settled?
@captain_mom7 asks: What took us so long to get this picture? Why is this hard? Physics major and Women in Physics Club co-president Mae Lee ’20 answers:
In short, this process took so long because of the sheer volume of data that had to be processed. To get a full picture of the black hole, we would need a satellite dish as large as the entire Earth! Instead, we have eight satellite locations that together make up the Event Horizon Telescope; they give us bits of the picture which we can feed through an algorithm that can piece together the rest of the image. The telescope at each location collected 64 gigabytes of data every second, which is around 1,000 times as fast as your standard home internet connection. This is too large to be transported over the internet, so it had to be physically transported (from all around the world to Massachusetts). In addition to transportation, that amount of data had to be fed through the algorithm, which also took a long time. These are just some of the reasons why it took so long to get this photo!
For the visual learners out there, Lee also answers this question in comic strip form.
On Facebook, Santosh Zachariah asks: Might it be possible one day to see a (false-color) rainbow around a black hole? In this image, since the electromagnetic radiation (3mm IR, I believe) from the accretion disk is bending around the black hole, much like visible light in a raindrop, would the dimensions of the image have been different at a different wavelength? And if so, what might a black-hole-bow look like?
Professor Shuve answers: That’s a great question! The short answer is: No, different wavelengths of life wouldn’t bend at different angles, BUT light originating from different distances from the black hole center could arrive in different colors. Let me explain a bit more.
In our current theory of gravity, general relativity, the force of gravity is actually a manifestation of the bending of space and time. Every object is trying to go in as straight of a line as possible, but the bending of spacetime means that objects tend to have paths that converge over time (which is gravitational attraction). A consequence of this is that every object is attracted identically under gravity: Because the gravitational force is really just objects moving through a curved spacetime, every object bends the same way when travelling through that spacetime. So different colors (or wavelengths) of light will bend the same way due to the gravitational force.
However, there is an additional effect called “redshift.” Essentially, light that originates from closer to the black hole has to expend more energy to escape the black hole and get to us than light emitted further from the black hole. In quantum mechanics, light with lower energy is redder in color (has a longer wavelength), so that means that light from closer to the black hole will appear as different in color. This means that if we had sources that emitted a single color (wavelength) of light, but some were closer than others to the black hole, we would see a combination of different colors coming out at us. In practice, the light is being emitted at a lot of different wavelengths, so we wouldn’t see anything like a rainbow, but the color of the light is nevertheless changing between its emission near the black hole and the telescopes on Earth.